Quantum Computing’s Next Chapter: The Willow Chip and the Future of Security
- Dec 13, 2024
- 4 min read
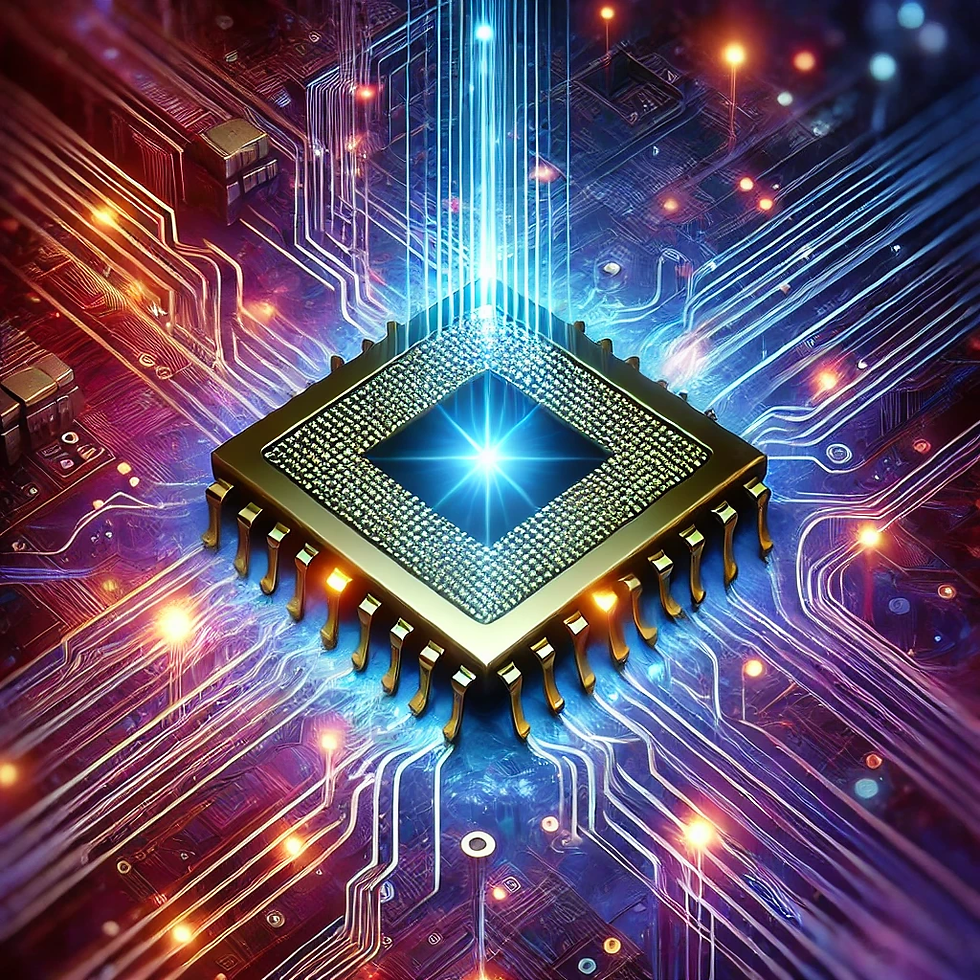
For years, quantum computing has been the epitome of technological futurism, offering glimpses of solving previously insurmountable problems. However, as artificial intelligence (AI) surged to dominate headlines, quantum computing temporarily receded from public focus. Enter Google’s Willow quantum computing chip, a breakthrough that reignites interest in quantum technologies and their far-reaching implications—not just for computation but also for cybersecurity.
While AI advancements are reshaping industries, quantum computing, with the power of chips like Willow, promises to redefine the landscape of cryptography and data security. This blog explores how Willow positions quantum computing at the forefront of cybersecurity, ushering in the age of quantum encryption algorithms.
Willow: A Quantum Leap Forward
Google’s Willow chip is a quantum processor with 105 qubits, engineered to solve problems far beyond the capacity of classical computers. Unlike a standalone quantum computer, Willow acts as the computational heart, driving quantum systems to unprecedented performance. Notably, the chip has demonstrated the ability to complete computations in minutes that would take classical supercomputers longer than the age of the universe.
This milestone is a testament to the practical viability of quantum computing and underscores its potential to tackle challenges such as climate modeling, AI optimization, and cybersecurity.
The Cybersecurity Paradox
Quantum computing introduces both solutions and threats to modern cryptography. Its immense power enables breakthroughs in data security but also raises alarms about the vulnerability of current encryption standards.
1. Fighting Ransomware: The Positive Side
Ransomware attacks, which encrypt victims’ data and demand payment for the decryption key, could be neutralized by quantum computing:
Most ransomware relies on encryption algorithms such as RSA or ECC, which depend on the computational difficulty of factoring large numbers or solving discrete logarithms.
With Shor’s algorithm, a quantum system powered by Willow can solve these problems exponentially faster. For instance:
Factoring a 2048-bit RSA key might take trillions of years on a classical computer but could be accomplished in hours with a sufficiently advanced quantum system.
This would allow authorities to decrypt ransomware-locked data without paying attackers, potentially rendering this form of cybercrime obsolete.
2. Breaking Encryption: The Negative Side
Conversely, the same quantum capabilities can undermine the very encryption standards that protect sensitive data:
Public-key cryptographic systems like RSA and ECC rely on problems that are trivial for quantum systems to solve.
Imagine a scenario where a quantum computer breaks into encrypted financial records or classified government data in minutes, threatening national security and individual privacy.
Even today, attackers could engage in "harvest now, decrypt later" schemes, collecting encrypted data for future decryption once quantum capabilities are available.
The Dawn of Quantum Encryption Algorithms
Recognizing the existential threat posed by quantum computing to traditional cryptography, the cybersecurity community is racing to develop quantum-resistant algorithms. These efforts fall into two main categories: Post-Quantum Cryptography (PQC) and Quantum Key Distribution (QKD).
Post-Quantum Cryptography
PQC involves classical cryptographic algorithms designed to withstand attacks from both classical and quantum computers. Key examples include:
CRYSTALS-Kyber: A lattice-based algorithm for general encryption.
CRYSTALS-Dilithium: A lattice-based algorithm for securing digital signatures.
SPHINCS+: A hash-based algorithm for digital signatures.
Unlike traditional encryption, PQC relies on mathematical problems like lattice cryptography, which are resistant to quantum attacks.
Quantum Key Distribution
QKD leverages quantum mechanics to secure communications. Using principles like entanglement, QKD enables two parties to share encryption keys securely. If an eavesdropper attempts to intercept the communication, the quantum state of the system changes, alerting the parties to the intrusion. While promising, QKD requires specialized infrastructure and is not yet widely adopted.
Implications for AI Processing
Beyond cybersecurity, quantum computing promises significant advancements in AI processing:
Optimization: Quantum chips like Willow excel at solving optimization problems critical to AI, such as training large neural networks.
Parallelism: Quantum computers process massive datasets simultaneously, reducing bottlenecks in machine learning workflows.
Hybrid Systems: Combining classical and quantum computing could revolutionize fields like natural language processing and predictive modeling.
For instance, a quantum-assisted AI model could analyze billions of data points in real-time, offering unprecedented insights into global markets, healthcare, or climate science.
Mathematical Insight: Shor’s Algorithm
The ability of quantum computers to break traditional encryption lies in algorithms like Shor’s:
Classical factorization of a 2048-bit RSA key takes:
This means the time grows exponentially with the cube root of the logarithm of n, where n is the number to be factored. For large n, this growth becomes astronomically large. Even the most powerful classical supercomputers would require billions of years to factor a 2048-bit key.
Quantum factorization reduces this to:
This means that a task infeasible for even the most powerful classical supercomputers becomes practical for a quantum system. The time grows only as the cube of the logarithm of n. This growth is far slower than the exponential growth in the classical case.
This disparity means that quantum computers can solve problems in hours or minutes that classical computers would take trillions of years to solve. This isn’t just a speed improvement—it’s an entirely new level of computational capability. Tasks like breaking modern encryption, which are impossible with classical computers, become routine with quantum systems.
Preparing for the Quantum Era
The transition to quantum-resistant systems is not optional; it is a necessity. Governments, corporations, and cybersecurity firms must:
Adopt PQC Standards: Algorithms like CRYSTALS-Kyber and Dilithium must replace traditional encryption schemes.
Implement Hybrid Systems: Use a combination of classical and quantum techniques for robust security.
Secure Data Today: Encrypt sensitive information with quantum-resistant methods to preempt "harvest now, decrypt later" attacks.
Conclusion
Google’s Willow chip represents a defining moment in quantum computing, bringing the technology from theoretical promise to practical application. While it offers unprecedented capabilities to counteract threats like ransomware, it simultaneously demands a complete overhaul of modern encryption standards.
The age of quantum computing is here, and with it comes the necessity for quantum encryption algorithms and a global pivot to quantum-resilient systems. As we stand at the intersection of quantum computing and AI, the transformative potential of these technologies is undeniable—but so too are the challenges we must overcome to secure the future.
#QuantumComputing #CyberSecurity #AIRevolution #GoogleWillow #QuantumEncryption #PostQuantumCryptography #RansomwareDefense #ShorsAlgorithm #QuantumBreakthrough #EncryptionFuture #AIandQuantum #QuantumCryptography #DataSecurity #FutureOfTech #TechInnovation #QuantumVsClassical #QuantumAI #SecureFuture #CryptographyRevolution #QuantumEra
Comments